Fertilization
The process of fertilization differs considerably between animal groups but there are a couple of common features. When the sperm fuses with the egg there's a reasonably rapid change in egg structure that excludes the fusion of any longer sperm. This is often called a block to polyspermy. Fusion activates the inositol trisphosphate signal transduction pathway leading to a rapid increase in intracellular calcium. This causes exocytosis of cortical granules whose contents form, or contribute to, a fertilization membrane; and also triggers the metabolic activation of the egg, increasing the speed of protein synthesis and, in vertebrates, starting the second meiotic division. The calcium may, additionally, trigger cytoplasmic rearrangements that position determinants that are important for the longer-term regional specification of the embryo. For instance, dorsal localization of components of the Wnt pathway in Xenopus, or polar granule segregation in C. elegans occurs during this manner. The sperm and egg pronuclei fuse to make one diploid nucleus and at this stage, the embryo is understood as a zygote.
Cleavage
A typical zygote of an animal embryo is little, spherical, and polarized along the vertical axis. The upper hemisphere, usually carrying the polar bodies, is named the animal hemisphere, and therefore the lower hemisphere, rich in the yolk, the vegetal hemisphere. the first cell divisions are called cleavages. They differ from normal cellular division therein there's no growth phase between successive divisions. So each division partitions the cell into two half-size daughters . The products of cleavage are called blastomeres. Cellular division without growth can proceed for a substantial time in free-living embryos without an extracellular yolk mass. Embryos that do have some sort of food supply, either mammals that are nourished by the mother or egg types with an outsized yolk mass like birds and reptiles, only undergo a limited period of cleavage at the start of development. In many species, the embryo’s own genome remains inactive during part or all of the cleavage phase, and protein synthesis is directed by messenger RNA transcribed during oogenesis (maternal mRNA). This is often the stage of genetic maternal effects because the properties of the cleavage stage embryo depend entirely on the genotype of the mother and not thereon of the embryo itself.
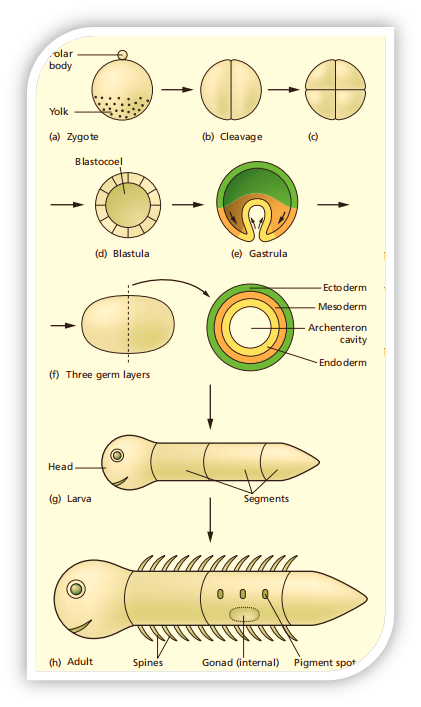
Different animal groups display different types of cleavage and this is often controlled to an outsized extent by the quantity of yolk within the egg. Where there's tons of yolk, as in an avian egg, the cytoplasm is concentrated near the animal pole and only this region cleaves into blastomeres, with the most yolk mass remaining acellular. This sort of cleavage is named meroblastic. Where cleavage is complete, dividing the entire egg into blastomeres, it's called holoblastic. Holoblastic cleavages are often somewhat unequal, with the blastomeres within the yolk-rich vegetal hemisphere being larger (macromeres), while those within the animal hemisphere are smaller (micromeres). Each animal class or phylum tends to possess a characteristic mode of early cleavage and these are often classified by the arrangement of the blastomeres into such categories as radial (echinoderms), bilateral (ascidians), and rotational (mammals). A crucial type is that the spiral cleavage shown by most annelid worms, molluscs, and flatworms. Here, the macromeres stop successive tiers of micromeres, first during a right-handed sense when viewed from above, then another tier during a left-handed sense, and so on. Most insects and a few crustaceans show a special sort of cleavage called superficial cleavage. Here only the nuclei divide and there's no cytoplasmic cleavage at the first stages. Thus, the first embryo becomes a syncytium consisting of the many nuclei suspended within an equivalent body of cytoplasm. At a particular stage, the nuclei migrate to the periphery and shortly afterwards cell membranes grow in from the outer surface of the embryo and surround the nuclei to make an epithelium.
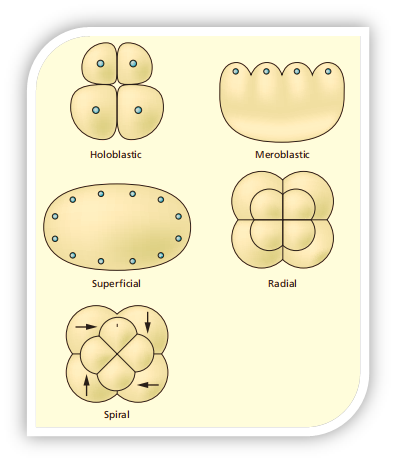
During the cleavage phase, a cavity usually forms within the centre of the ball of cells, or a sheet of cells within the case of meroblastic cleavage. This expands thanks to the uptake of water and becomes referred to as the blastocoel. At this stage of development, the embryo has named a blastula or blastoderm. The cells often adhere tightly to at least one another, being bound by cadherins, and can usually have a system of tight junctions forming a seal between the external environment and therefore the internal environment of the blastocoel.
Gastrulation
The germ cells have usually appeared by the stage of gastrulation and aren't considered belonging to any of the three germ layers. After the completion of the main body morphogenetic movements, most sorts of the animal embryo have reached the overall body plan stage at which each major part is present as a neighbourhood of committed cells, but is yet to differentiate internally. This stage is usually called the phylotypic stage, because it's the stage at which different members of a biological group, not necessarily an entire phylum, show maximum similarity to every other. For instance, all vertebrates show a phylotypic stage at the tailbud stage once they have a notochord, ectoderm, paired somites, branchial arches, and tailbud. All insects show a phylotypic stage at the extended germband once they show six head segments, three appendage bearing thoracic segments, and a variable number of abdominal segments.
The germ cells have usually appeared by the stage of gastrulation and aren't considered belonging to any of the three germ layers. After the completion of the main body morphogenetic movements, most sorts of the animal embryo have reached the overall body plan stage at which each major part is present as a neighbourhood of committed cells, but is yet to differentiate internally. This stage is usually called the phylotypic stage, because it's the stage at which different members of a biological group, not necessarily an entire phylum, show maximum similarity to every other. For instance, all vertebrates show a phylotypic stage at the tailbud stage once they have a notochord, ectoderm, paired somites, branchial arches, and tailbud. All insects show a phylotypic stage at the extended germband once they show six head segments, three appendage bearing thoracic segments, and a variable number of abdominal segments.
Axes and symmetry
In order that specimens are often oriented in a consistent way, it's necessary to possess terms for describing embryos. If the egg is approximately spherical with an animal and vegetal pole then the road joining the 2 poles is that the animal–vegetal axis. Unfertilized eggs are usually centrosymmetric around this axis, but after fertilization, there's often a cytoplasmic rearrangement that breaks the initial symmetry and generates a bilaterality. In some organisms, like Drosophila, this might occur earlier, within the oocyte; in others, like mammals, it's going to occur later, at a multicellular stage. But even animals like sea urchins, which are centrosymmetric as adults, or gastropods, which are asymmetrical as adults, still have bilaterally symmetrical early embryos. The change of symmetry means the animal now features a distinct dorsal (upper) and ventral (lower) side.
If the animal and vegetal poles are at the highest and bottom, then the equatorial plane is that the horizontal plane dividing the egg into animal and vegetal hemispheres, a bit like the equator of the world. Any vertical plane, like circles of longitude, is named a meridional plane. Once the embryo has acquired its bilaterality then there's a very meridional plane at right angles to the median plane and is usually, but not necessarily, the plane of the second cleavage.
Following gastrulation most animals become elongated. the top end is that the anterior, the tail end is that the posterior, therefore the head-to-tail axis is named anteroposterior. The top-bottom axis is named dorsoventral and therefore the left-right axes are called mediolateral. In human anatomy, because we stand upright on two legs, the term anteroposterior is generally synonymous with dorsoventral, but the term “craniocaudal” remains acceptable for the head-to-tail axis. The terms proximal and distal are usually utilized in reference to appendages, proximal meaning “near the body” and distal meaning “further faraway from the body.”
Generally, the principal body parts will appear a while after the completion of gastrulation. Some phyla, including annelids, arthropods, and chordates, show prominent segmentation of the anteroposterior axis. To qualify as segmented an organism should show repeated structures that are similar or just like one another, are principal instead of minor body parts, and involve contributions from all the germ layers. Although most animals have an overriding bilaterality, this is often not exact and there are systematic deviations that make right and left sides slightly different. for instance in mammals the cardiac apex, stomach, and spleen are on the left and therefore the liver, vena cava, and greater lung lobation are on the proper. This asymmetrical arrangement is understood as situs.
If the arrangement is inverted, as occurs in some mutants or experimental situations, it's called situs inversus. If the parts on the 2 sides are partly or wholly equivalent, it's called isomerism.
Developmental control genes
The state of commitment of various body parts is controlled by the expression of specific sets of developmental control genes. These are sometimes called homeotic genes, or selector genes. The expression of those genes in embryos is controlled by cytoplasmic determinants or by inducing factors.
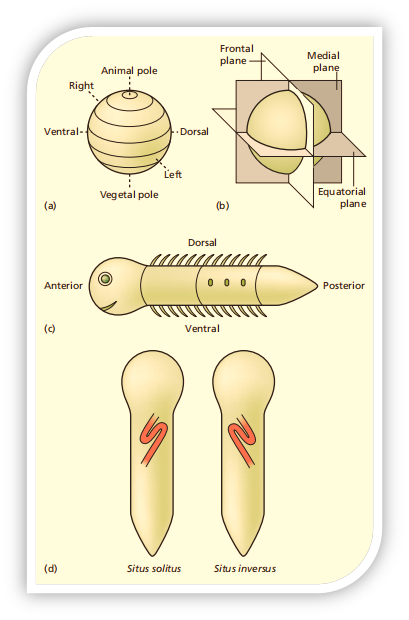
Developmental control genes virtually all encode transcription factors, whose function is regulating the activity of other genes. It's important to notice that for such genes the maximum amount of information is encoded by the “off” state as by the “on” state because the absence of a repressor is often like the presence of an activator. The existence of two discrete states of gene activity may be a natural way of ensuring a pointy and discontinuous threshold response to a determinant or inductive signal. a method of ensuring that there are just two discrete states of activity for developmental control genes is to possess a positive feedback regulation. This sort of system is named a bistable switch because it's two stable states. The gene is off if both the regulator and therefore the gene product are absent. it's initially turned on by the regulator, which could be a cytoplasmic determinant or a sign transduction pathway activated by an inducing factor. Once the gene product has accumulated, the gene remains on albeit the regulator is removed. This model shows three critically important features of gene regulation in development.
Firstly, it can yield a pointy and discontinuous threshold in response to the regulator. Secondly, the system has a memory of exposure to the regulator. this is often because the gene remains on permanently despite its transient exposure to the regulator. Thirdly, bistable switches are kinetic phenomena. this suggests that they depend upon the continual production and removal of drugs, during this case the gene product. the straightforward equilibrium thermodynamic properties of binding and dissociation cannot create sharp thresholds or memory. Although sharp thresholds of gene activity are quite common, they're in most cases maintained by far more complex mechanisms than this easy positive-feedback loop and involve many gene products.

Morphogen gradients
The properties of inductive signals explain how it's possible for an embryo to extend in complexity. There must be a minimum of two regions to start with: one emitting the signal and therefore the other responding thereto. Albeit there's only one threshold response to the signal, the responding tissue is going to be partitioned into two territories so there's a rise of territories from two to 3. Often, however, there's quite one threshold response to an inducing factor, at different concentrations of the signal. If the inducing factor is distributed during a concentration gradient then multiple threshold responses can cause the formation of a posh pattern in one step. Such a gradient controls the kinds of territories, their sequence in space, and therefore the overall orientation or polarity of the series of latest structures. When inducing factors are present as gradients with multiple responses, they're called morphogens.
A stable concentration gradient can't be produced just by releasing a pulse of the morphogen. Such a pulse would open up by diffusion and eventually the concentration would become uniform everywhere the embryo. a degree gradient is merely found out during a situation where the morphogen is continuously produced in one region (a source) and destroyed in another (a sink). This may produce a gradient of concentration, with a flux of fabric from the source to the sink. A model that seems to suit the behaviour of the many natural systems maintains a continuing concentration of morphogen within the signalling region and has destruction at a rate proportional to local concentration throughout the responding tissue. This produces a gradient that is approximate of exponential form when it reaches the steady-state.
A concentration gradient has two important properties. It can subdivide the competent zone of cells into several states of commitment by means of threshold responses, and it automatically imparts a polarity also as a pattern to the responding tissue. The competent field of cells is subdivided into four territories by the activation of three developmental control genes during a nested pattern. Because there are just the 2 states of gene activity, they're represented by binary digits where “1” means “on” and “0” means “off.” The action of the gradient will subdivide the sector into four territories (here head and three body segments) with the codings 000, 001, 011, 111. within the second example, suppose that graft has been administered to put a second source at the opposite end of the sector. With a source at both ends and destruction throughout the central region, the concentration gradient will become U-shaped. An equivalent threshold responses will now produce a special pattern 111, 011, 001, 011, 111. this is often a kind of structure called a mirror-symmetrical duplication because it consists of two similar halves joined by a plane of parity. The polarity is normal within the right half but inverted within the left half of the sector. Mirror-symmetrical duplications arise very often in embryological experiments following the grafting of signalling centres, for instance, the double-dorsal Xenopus embryo arising from the creation of a second organizer, or the double posterior limbs produced by ZPA grafting. Within the lower panel is shown another sort of experiment involving the insertion of an impermeable barrier that interrupts the passage of the morphogen. Because no morphogen is being produced on the left-hand side, the concentration soon falls to zero, while on the right-hand side it actually piles up to a better concentration than normal. This is often because the dimensions of the sink have been reduced relative to the source. Because the rate of destruction is proportional to concentration, the general concentration has got to increase to re-establish the steady state. Operation of an equivalent threshold responses shows that not only has the left half of the pattern been lost, but so has a part of the proper half because the elevation of concentration has expanded the dimensions of the foremost posterior territories. this instance shows how the properties of developmental systems might not be obvious initially sight. They'll sometimes be countered intuitive, and interpretation of experimental results may require an understanding of the properties of the underlying dynamic mechanisms also as a familiarity with the genes and signalling
molecules at work.
Homeotic mutations
A homeotic mutation is one that will convert one part into another. How this will happen is explained by our simple model. Suppose that the organism may be a mutant in which the second gene within the series is permanently inactive and can't be turned on. During this case, the codings are going to be 000, 001, 001, and 101. In other words, the second body segment has now been becoming another copy of the primary body segment. What happens to the third, tail, segment can't be predicted without knowing more about the logical circuitry, because this now features a novel coding not found within the normal organism. this instance illustrates the behaviour of a loss-of-function homeotic mutation. Such mutations are genetically recessive because function would be restored by an honest copy of the gene on either chromosome.

On the opposite hand, suppose that the mutation in gene 2 caused constitutive activity, in other words, the gene is on all the time everywhere. Now the sequence of codings would become 010, 011, 011, 111. Here the primary body segment has become a replica of the second body segment and therefore the head has an abnormal coding. This is often a gain-of-function mutation. It's genetically dominant because inappropriate activation will occur with just one copy of the mutated gene. this instance represents, during a highly oversimplified manner, the operation of the Hox gene system for anteroposterior patterning of animals. Generally, loss-of-function mutations produce interiorization, and gain-of-function mutations produce posteriorizations. It also illustrates the combinatorial nature of regional specification in development. Although striking anatomical transformations may sometimes be produced by the manipulation of individual genes, the identity of body parts is really controlled in normal development by combinations of several genes.
Homeotic, homeobox, and Hox
Considerable confusion arises about the connection between the definition of homeotic behaviour and therefore the molecular identity of homeotic genes. In 1984, a standard DNA sequence was discovered within the genes of the Bithorax and Antennapedia complexes of Drosophila. Because these genes were homeotic, this motif was called the “homeobox.” The proteins encoded by these genes are all transcription factors and therefore the homeobox encodes a sequence of 60 basic amino acids which form their DNA binding domain, called the homeodomain. Subsequently, many homeobox-containing genes were found altogether sorts of eukaryotic organisms. All homeobox-containing genes encode homeodomain-containing transcription factors. Many, but not all, of those are concerned with development, but rather few of them are homeotic when mutated or misexpressed. The Hox genes are a family of genes, found in animals but not in other eukaryotes, that are liable for specifying anteroposterior identity to body levels. they're a subset of the overall class of homeobox genes and are specifically the homologues of the Drosophila Bithorax and Antennapedia gene clusters. In many animals, the Hox genes from one gene cluster, with the various genes adjacent to every other on the chromosome.
The Hox genes are activated at an early stage in body-plan formation and are generally maximally expressed around the phylotypic stage of the group in question. Each gene within the cluster is expressed at a specific anteroposterior level, running from a pointy anterior boundary to dissolve gradually within the posterior. they're expressed in both central systema nervosum and mesoderm. Remarkably, the spatial order of expression of Hox genes within the body, from anterior to posterior, is usually equivalent because of the order of the arrangement of the genes on the chromosome. Invertebrates have only one cluster of Hox genes but vertebrates have four or more clusters, each situated on a special chromosome.
References :
Essential Developmental Biology by Jonathan M.W. Slack.
Comments